Archive
Synthetic biology and living processors
As crazy and sci-fi as it might seem to think of building a computer using cellular components, it was even more shocking to me to learn that the term, “synthetic biology” has a history that goes all the way back to 1910. In fact, in 1974, Polish geneticist Wacław Szybalski used the term to examine the idea of using molecular biology in a synthetic manner to “devise new control elements” in a modular manner for creating new genomes, new organisms, etc… BRILLIANT!!! It took some time for technology — and science — to catch up with such revolutionary thinkers, and synthetic biology finally began to take off in 2000.
Really, the idea of synthetic biology (horribly over-simplified) is based on the recognition that biological organisms — no matter how simple — process their environment remarkably quickly, with a resolution and speed beyond anything we, as engineers, have been capable of achieving so far. Another way to think about this is that in this modern age of BIG DATA, for which our greatest challenges are how to store, manage, and analyze the continuous onslaught of exabytes (hunh??) of data, biological organisms had this figured out… well… millions of years ago. Youtube’s streaming video? Got it! Bose’s Quiet Comfort noise canceling earphones? Remember how we all ignored nagging parents or droning lecturers… oh yes, got that. iPhone’s accelerometers? Yup. And what’s even more inspirational or frustrating (depending on which side of the technological line you fall) is that we don’t only do it faster, we do it better.
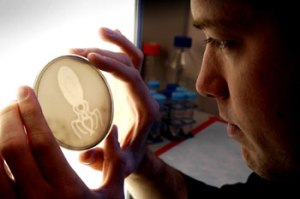
Then UT Austin Ph.D. student Jeff Tabor (now a prof at Rice University), holding a bacteria-produced photo of an enlarged E. coli bacterium…
Photo by: Marsha Miller, UT Austin
Here’s an example. In 2004, in response to a synthetic biology competition call by the International Genetically Engineered Machine (iGEM) Foundation, a group of students at UT Austin invented a method for making photos out of… bacteria! The basic idea is that they genetically-engineered E. coli bacteria to respond to light, giving them a new biological circuit that would cause them to turn black when growing in dark areas and to turn clear in the light. A reasonable analogy would be to think of each bacterium as a pixel on your computer or TV screen. By then spreading and growing them evenly on a Petri dish as a homogeneous lawn of bacteria, and then projecting lighted images on them, the students could reproduce images using these genetically-engineered bacteria.
Why is this exciting? Well, for all of you owners of the latest iPad, iPhones, and MacBook Pros, your stunningly gorgeous Retina displays have approximately 326 PPI (that stands for “pixels per inch”). In contrast, with the bacterial images developed in 2004 (yes, nine years ago!), we’re talking gigapixels per square inch resolution, or thousands of pixels per inch, to make it more comparable to the PPI unit. That’s an order of magnitude greater than the Retina displays… I mean… WOW!
But, ok. So for all of you super-skeptics out there, why should we care? This is a monochromatic display that can (literally) die, when we’re into vibrant, saturated, archival colors seamlessly (sort of) integrated into our electronics… not to mention that these students had to build a seven-foot tall projector to create these relatively simple-looking images… which don’t even move! (Yes, we can all hear the collective gasp by the MTV generation and onwards.) [A side note to all you curmudgeons: I still think this is wonderfully cool.]
Let’s get back to the inspiration for this post. Last week, the journal Nature published an article by Daniel and colleagues from MIT, titled: “Synthetic analog computation in living cells”. While our obsession with the digital processing universe makes this sound like a step back into the Dark Ages, an advantage of analog devices is that they can be simpler while maintaining greater bandwidth and frequency range (yes, think about this from the standpoint of music!). When a signal is converted from analog to digital, it can lose some of its range (or fidelity) depending on the sensors involved, and this conversion process takes time.
Some of you may have heard of the analogy of a biological cell being somewhat like a digital computer, which processes everything as a series of ones and zeros. In reality, however, this is actually a gross oversimplification. While cells do respond to certain stimulants with a binary (on/off) response, the reality is that they use a mix of these digital-like responses and graded responses (think grays, and sorry, I’m not referring to Fifty Shades of Gray) when responding to various inputs — something the digital world of ones and zeros, black and white, cannot do. However, those who have tried to take the cell-computer analogy all the way have been able to use DNA as components for producing digital calculators, sensors and the like. The drawback is that these circuits require an enormous number of components to perform the simplest computations. Each component could be a DNA strand or a protein, which makes the process slower and more difficult to reproduce.
The system that Daniel and colleagues have assembled takes bacterial cells and transforms them into living calculators that can compute logarithms, multiplication, division, and can perform even more sophisticated functions such as acting as an in vivo pH meter… with three or fewer genetic parts. Furthermore, because their system operates in the analog signal processing domain, it can process graded information, characteristic of the natural environment in which we live and with which we interact. Such analog computation could permit the design of cellular sensors for pathogens or toxins. It may now also be possible to combine their analog technology with the digital systems to construct a synthetic digital/analog hybrid system that can swap between the two signal processing approaches according to which mechanism can produce faster or more accurate calculations or processes. Also, from the broader biological context, it now may also be possible to observe the behavior of such a synthetic, analog system and begin to gain a better understanding for how biological systems receive and process complex information, permitting the rapid responses and fine control necessary to make life a brilliant, rapid, high-resolution reality.